Make: Electronics by Charles Platt (read me a book .TXT) π
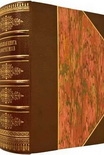
Read free book Β«Make: Electronics by Charles Platt (read me a book .TXT) πΒ» - read online or download for free at americanlibrarybooks.com
- Author: Charles Platt
Read book online Β«Make: Electronics by Charles Platt (read me a book .TXT) πΒ». Author - Charles Platt
D1, D2: Light-emitting diodes
S1: DPDT relay
S2: SPST momentary switch
C1: Electrolytic capacitor, 1,000 Β΅F
R1: Resistor, 680Ξ© minimum
Figure 2-75. If your breadboard doesnβt have screw terminals, insert two short pieces of solid-core wire with stripped ends and then attach the stranded wires from the adapter using alligator clips.
Youβll need some more 22-gauge wire, or some precut hookup wire, to supply the power to your components, which are plugged into the breadboard as shown in Figures 2-76 and 2-77. If you get all the connections right, the circuit should function the same way as before.
The geometry of the metal connecting strips in the breadboard often forces you to connect components in a roundabout way. The pushbutton, for instance, supplies power to the pole of the relay but cannot be connected directly opposite, because there isnβt room for it.
Remember that the strips inside the breadboard that donβt have any wires or components plugged into them are irrelevant; they donβt do anything.
Iβll include some suggested breadboard layouts for circuits as you continue through this book, but eventually youβll have to start figuring out breadboard layouts for yourself, as this is an essential part of hobby electronics.
Figure 2-76. Two oversized LEDs, one resistor, and the necessary jumper wires have been added to the breadboard.
Figure 2-77. Now the pushbutton, relay, and capacitor have been added to complete the circuit shown in the diagram and the schematic. When the pushbutton is pressed, the relay oscillates and the LEDs flash.
Experiment 9: Time and Capacitors
You will need:
AC adapter, breadboard, wire, wire cutters, and strippers.
Multimeter.
Pushbutton, SPST. Quantity: 1.
Resistors and electrolytic capacitors, assorted.
In Experiment 8, when you put a capacitor in parallel with the coil of the relay, the capacitor charged almost instantly before discharging itself through the relay coil. If you add a resistor in series with a capacitor, the capacitor will take longer to charge. By making a capacitor take longer to charge, you can measure time, which is a very important concept.
Clean the components off your breadboard and use it to set up the very simple circuit shown in Figure 2-78, where C1 is a 1,000 Β΅F capacitor, R1 is a 100K resistor, R2 is a 100Ξ© resistor, and S1 is the pushbutton that you used previously. Set your meter to measure volts DC, place the probes around the capacitor, and hold down the pushbutton. You should see the meter counting upward as the voltage accumulates on the capacitor. (This is easier with a meter that doesnβt have autoranging, because you wonβt have to wait while the meter figures out which range to apply.) Resistor R1 slows the charging time for the capacitor.
Figure 2-78. Watch the voltage building up on the capacitor while you hold down the pushbutton. Substitute other values for R1, discharge the capacitor by touching R2 across it, and then repeat your measurement process.
S1: Momentary pushbutton, OFF (ON)
R1: 100K initially
R2: 100Ξ©
C1: 1,000 Β΅F
Release the pushbutton, set aside your meter, and discharge the capacitor by touching R2 across it for a second or two. Now substitute a 50K resistor for R1, and repeat the measurement. The meter should count upward almost twice as fast as before.
Voltage, Resistance, and Capacitance
Think of the resistor as a faucet, and the capacitor as a balloon that you are trying to fill with water. When you screw down the faucet until only a trickle comes through, the balloon will take longer to fill. But a slow flow of water will still fill the balloon completely if you wait long enough, and (assuming the balloon doesnβt burst) the process ends when the pressure inside the balloon is equal to the water pressure in the pipe supplying the faucet. See Figure 2-79.
Figure 2-79. When the faucet is closed half-way, the balloon will take longer to fill, but will still contain as much water and as much pressure in the end.
Similarly, in your circuit, if you wait long enough, eventually the voltage across the capacitor should reach the same value as the voltage of the power supply. In a 12-volt circuit, the capacitor should eventually acquire 12 volts (although βeventuallyβ may take longer than you think).
This may seem confusing, because earlier you learned that when you apply voltage at one end of a resistor, you get less voltage coming out than you have going in. Why should a resistor deliver the full voltage when it is paired with a capacitor?
Forget the capacitor for a moment, and remember how you tested just two 1K resistors. In that situation, each resistor contained half the total resistance of the circuit, so each resistor dropped half the voltage. If you held the negative probe of your meter against the negative side of your power supply and touched the positive probe to the center point between the two resistors, you would measure 6 volts. Figure 2-80 illustrates this.
Now, suppose you remove one of the 1K resistors and substitute a 9K resistor. The total resistance in the circuit is now 10K, and therefore the 9K resistor drops 90% of the 12 volts. Thatβs 10.8 volts. You should try this and check it with your meter. (You are unlikely to find a 9K resistor, because this is not a standard value. Substitute the nearest value you can find.)
Now suppose you remove the 9K resistor and substitute a 99K resistor. Its voltage drop will be 99% of the available voltage, or 11.88 volts. You can see where this is heading: the larger the resistor, the larger its contribution to voltage drop.
However, I noted previously that a capacitor blocks DC voltage completely. It can accumulate an electrical charge, but no current passes through it. Therefore, a capacitor behaves like a resistor that has infinite resistance to
Comments (0)